What are extreme environments?
- An extreme environment is a habitat characterized by harsh environmental conditions further than the optimal range for the development of humans or other living organisms.
- Extreme environments are characterized by various unfavorable conditions including, high or low temperature, high or low pressure, and acidic or basic pH.
- For an area to be considered extreme, certain conditions or aspects of the environment must be considered very hard for different forms of life to survive.
- Examples of some extreme environments include the polar region, deserts, volcanic regions, deep ocean trenches, outer space, and every other planet of the Solar System except the Earth.
- Some of the common extreme environments include areas that are alkaline, acidic, extremely hot or cold, high salt concentration, without water or oxygen.
Extreme environments are classified into the following groups based on the extreme physicochemical conditions:
Extreme temperature: Two types of extreme environments can be described; cold and hot.
- Extremely cold environments are those with environmental temperatures below 5°C. These can be found in deep ocean niches, at the peaks of high mountains, or the Polar Regions.
- Extremely hot environments are characterized by environmental temperatures higher than 45°C. These environments are influenced by geothermal activity as geysers and fumaroles of continental volcanic areas or deep-sea vents.
Extreme pH: Extreme environments can also be classified as acidic or alkaline according to their pH.
- Extreme acidic environments are natural habitats in which the pH is below 5.
- Extreme alkaline environments are those with a pH above 9.
Extreme ionic strength:
- Hypersaline environments are environments with an ionic concentration higher than of seawater (greater than 3.5%).
Extreme pressure:
- Extreme pressure environments are those environments under extreme hydrostatic or litho pressure, such as aquatic habitats at depths of 2,000 m or more or deep-subsurface ecosystems.
High-radiation environments are those areas that are exposed to abnormally high radiation doses, including ultraviolet or gamma radiation, like deserts and the top of high mountains.
Xeric environments are arid habitats with limited water activity. Cold and hot deserts are some examples of these extreme environments.
What are extremophiles?
- Extremophiles are living organisms with the ability to survive and thrive in extreme environments as a result of different physiological and molecular adaptations.
- These organisms thrive in extreme niches, ice, and salt solutions, as well as acid and alkaline conditions.
- Some might grow in toxic waste, organic solvents, heavy metals, or in several other habitats that are considered inhospitable for life.
- Most extremophiles are prokaryotic organisms with few eukaryotes. These extremophiles are defined by the environmental conditions in which they can survive and thrive optimally.
- Extremophiles can be divided into two categories: extremophilic organisms that require one or more extreme conditions to survive, and extremotolerant organisms that can tolerate extreme conditions of one or more physical parameters even though they grow optimally at neutral conditions.
- Extremophiles include members of all three domains of life; bacteria, archaea, and eukarya.
- Most extremophiles are prokaryotes with a high proportion of belonging to archaea, but some organisms might be eukaryotes such as protists (e.g., algae, fungi, and protozoa) and multicellular organisms.
- These are classified according to the conditions in which they grow: thermophiles and hyperthermophiles (organisms growing at high or very high temperatures, respectively), psychrophiles (organisms that grow at low temperatures), acidophiles and alkaliphiles (organisms thriving in habitats with acidic or basic pH values, respectively), barophiles (organisms that grow best under pressure), and halophiles (organisms that grow well in an environment with NaCl).
Characteristics of extremophiles
- Extremophiles are characterized by the ability to thrive in extreme environments which results from different forms of physiological and molecular adaptations.
- Extremophiles are mostly prokaryotes with the nuclear material in the cytoplasm and unicellular eukaryotes.
- Archea is an important group of organisms that tend to be extremophiles due to their ability to adapt to different extreme conditions.
- These organisms present a wide and versatile metabolic diversity, along with extraordinary physiological capacities to inhabit extreme environments.
- These forms of adaptations are developed through various evolutionary processes over a long period of time.
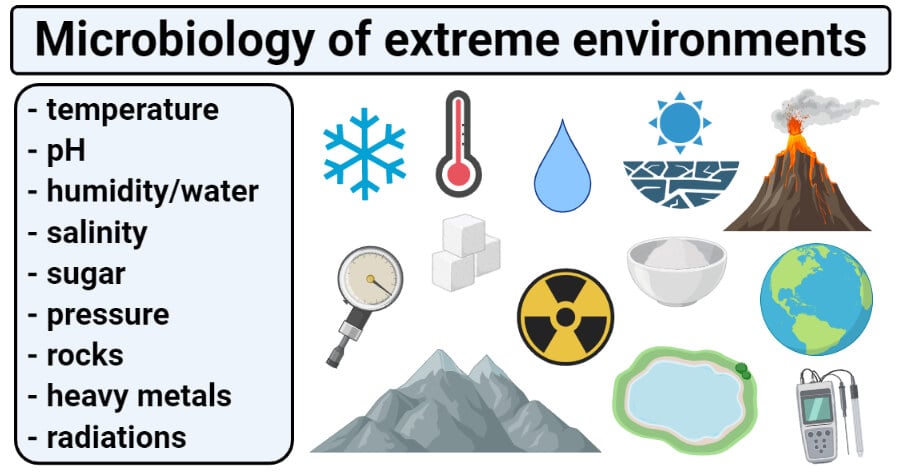
Microorganisms in extreme temperature (Psychrophile/Thermophile/Hyperthermophile)
Psychrophile Definition and Characteristics
- Psychrophiles, literally meaning cold-loving, are organisms adapted to growth at low temperatures, having an optimum growth temperature of greater than 15°C and a maximum growth temperature of greater than 20°C.
- Psychrophilic microorganisms have successfully colonized all permanently cold environments from the deep sea to mountain and polar regions.
- With more than 80% of Earth’s biosphere is permanently below 5°C, suitable environments for psychrophiles are widespread and include, among others, ocean waters, permafrost, glaciers, Antarctic rocks, snowfields, and polar ice caps.
- Some of these organisms, depending on their optimal growth temperature, are also known by the terms psychrotolerant or psychrotroph.
- Psychrophiles have successfully overcome two main challenges that arise during growth at low temperature: first, low temperature, because any decrease in temperature exponentially affects the rate of biochemical reactions; and second, the viscosity of aqueous environments.
- Prokaryotic psychrophiles vary in their requirement and indeed tolerance to oxygen and include (strictly) aerobic, (strictly) anaerobic, and facultative species.
- In addition to extremes of cold, many psychrophiles tolerate or in some cases require other extreme environmental conditions for growth and survival.
- Deep-sea psychrophiles, for example, may require high pressures for growth, thus making them barophilic psychrophiles.
Psychrophile Mode of adaptation
a. Proteins
- Psychrophiles produce proteins and enzymes that function optimally at cold temperatures.
- It has been observed that on average cold-active enzymes have a greater amount of α-helix and a lesser amount of β-sheet secondary structure.
- Because these β-sheets tend to form a more rigid structure, the α-helix content of cold-active enzymes allows the proteins greater flexibility in cold temperatures.
- Psychrophiles also produce antifreeze proteins that have the ability to bind to ice crystals through a large complementary surface and thereby create thermal hysteresis and lower the temperature at which an organism can grow.
- Another mechanism used to increase protein flexibility is by reducing the production of arginine and proline content that form multiple hydrogen bonds and salt bridges and reduce conformational flexibility.
b. Membrane
- The cytoplasmic membrane of psychrophiles contains a higher amount of unsaturated fatty acids which help to maintain a semi-fluid state of the membrane.
- Further adaptations that have been suggested to increase membrane fluidity include an increased content of large lipid head groups, proteins, and non-polar carotenoid pigments.
- The lipids of some psychrophiles also contain polyunsaturated fatty acids and long-chain hydrocarbons with multiple double bonds, which increase the fluidity of lipid membranes.
c. Genome structure
- The genomic structure of psychrophiles contains distinctly high G+C genomic regions, which mainly code for informational proteins like tRNAs, elongation factors, and RNA polymerases.
- Besides, a high level of redundancy occurs in the genomes of psychrophilic microorganisms, which encode multiple copies of tRNA species for the biosynthesis of all amino acids, as well as an increased variety and number of chaperones.
Psychrophile Examples
- Some common examples of psychrophiles include Psychrobacter members of Halomonas species, psychrophilic species of Pseudomonas and Alteromonas sps, Hyphomonas spp, Sphingomonas spp, Chryseobacterium greenlandensis, Desulfotalea psychrophila, Psychrobacter arcticus, etc.
Thermophile Definition and Characteristics
- Thermophiles (literally heat lovers) are organisms that grow at temperatures above those (25–40°C) that sustain most life forms. Typically, a thermophile shows maximum growth rates at temperatures above 45°C.
- There are many examples of the environment with extreme temperatures. Environments with high temperatures include both terrestrial and submarine environments.
- Different habitats throughout the world are suitable for the growth of thermophiles. These can range from surface soil to compost piles.
- Thermophiles are generally found in areas like thermal vents, hot springs, and boiling steam vents.
- Thermophiles are further divided into different groups; facultative thermophiles and obligate thermophiles.
- Facultative thermophiles can thrive at both high and moderate temperatures whereas obligate thermophiles require high temperature for growth.
- Like in the case of psychrophiles, thermophiles also have different physiological and molecular adaptations that enable the organisms to survive at temperatures that would normally denature proteins, cell membranes, and genetic material.
Thermophile Mode of adaptation
a. Proteins
- The enzymes and other proteins in these organisms are much more stable to heat than those in mesophiles.
- It is seen that a critical amino acid substitution on one or a few locations in the enzymes allows them to fold in such a way that it is consistent with heat stability.
- Besides, certain solutes such as diinositol phosphate and monosylglycerate are produced in significant quantities that help to stabilize the proteins against thermal degradation.
b. Cell membrane
- In addition to enzymes and other components of the cell, cytoplasmic membranes of thermophiles need to be stable.
- Thermophiles typically have lipid-rich in saturated fatty acids, thus allowing the membrane to remain stable and functional at high temperatures.
- Saturated fatty acids form a stronger hydrophobic environment than unsaturated fatty acids, which also increases membrane stability.
c. Nucleic acid
- Thermophiles contain special DNA binding proteins that arrange the DNA into globular particles that are more resistant to melting.
- Also, another factor that is common in all thermophiles is the presence of a unique DNA gyrase enzyme that acts to introduce positive supercoils in DNA, providing considerable heat stability.
Thermophile Examples
- Some of the common examples of thermophiles include Methanosarcina thermophila, Methanobacterium wolfei, Methanobacterium thermoautotrophicum, Archaeglobus profundus, Alicyclobacillus acidoterrestris, A. acidocaidarius, etc.
Hyperthermophile Definition and Characteristics
- Hyperthermophiles are organisms that can survive and grow at extremely high temperatures (above 80°C).
- Hypothermophiles are a type of thermophiles that can endure even higher temperatures than other thermophiles.
- The optimum temperature of growth for hyperthermophiles is 80°C, but they can survive at temperatures higher than 100°C.
- Most hyperthermophiles are capable of withstanding other extreme conditions like higher pH and higher pressure.
- Most hyperthermophilic organisms are found in hot springs and boiling steam vents where even moderate thermophiles cannot survive or thrive.
- Hyperthemophiles mostly belong to the archea group with very few species being bacterial species.
Hyperthermophile Mode of adaptation
a. Proteins
- Hyperthermophilic archaea used structure-based physical mechanisms to increase the thermostability of its proteins in the process of its thermophilic adaptation.
- The stability of proteins and enzymes in hyperthermophiles is improved as a result of an increased number of salt bridges (cations that bridge charges between amino acid residue) in the structure.
- Besides, an increase in the number of ionic bonds between the positive and negative charges of various amino acids also stabilizes proteins.
- The increased ionic bonds create a densely packed highly hydrophobic interior of the proteins, which resists the unfolding of proteins under high temperatures.
- Some hyperthermostable proteins even employ an alternative, sequence-based mechanism of their thermal stabilization.
b. Membranes
- Most hyperthermophilic archaea do not possess any fatty acids in their membranes; instead, they have lipids with branches hydrocarbon chains.
- These chains are composed of repeating units of a five-carbon compound, isoprene, bound to each other by ether linkages.
- The ether linkages are more stable linkages, which in turn, stabilizes the membranes against thermal breakage, and the branching reduces the membrane fluidity.
- The overall structure of membranes forms a lipid monolayer which is much more heat resistant than the lipid bilayer of mesophilic organisms.
c. Gene transfer
- DNA transfer is a vital part o several hyperthermophilic organisms in order to survive extremely high temperatures.
- Horizontal gene transfer takes place between species in the presence of high heat or UV radiation.
- Other processes like homologous recombination take place in some hyperthermophilic archaea in order to repair damaged DNA.
Hyperthermophile Examples
- Some of the common examples of hyperthermophilic organisms are Thermoproteus uzoniensis, Staphylothermus marinus, Pyrodictium abyssi, Pyrococcus furiosus, Hypothermus butylicus, Pryococcus woesei, Pyrodictium brockii, Pyrodictium occultum, etc.
Microorganisms in extreme pH (Acidophile/Alkaliphile)
Acidophile Definition and Characteristics
- Acidophiles are organisms that can survive and thrive at highly acidic conditions (usually at pH 2.0).
- Acidophilic microorganisms thrive in extremely low pH natural and man-made environments such as acidic lakes, some hydrothermal systems, acid sulfate soils, sulfidic regoliths, and ores, as well as metal and coal mine-impacted environments.
- Highly acidic environments are formed by the oxidation of the metal and other sulfidic minerals that are populated by a range of acidophilic and acid-tolerant prokaryotic and eukaryotic life forms.
- Heterotrophic, acidophilic bacteria, often living in close association with chemolithotrophic primary producers, have also been isolated from extremely acidic environments.
- The most widely studied acidophiles are prokaryotes that oxidize reduced iron and sulfur. They can catalyze the oxidative dissolution of metal sulfide minerals such as pyrite (FeS2), thereby severely acidifying the environment (often to pH less than 3) in which they thrive.
- There are several natural acidic environments that include volcanic areas, hydrothermal sources, deep-sea vents, metal mining areas, and the stomachs of animals.
- Acidophilic organisms belong to all three domains of life; archaea, bacteria, and eubacteria, but archaea represent the largest acidophilic group of organisms.
- Physiologically, the acidophiles are very diverse: aerobic and facultative anaerobic, chemolithotrophs, and different types of heterotrophic prokaryotes, photoautotrophic eukaryotes, predatory protozoa, and others.
Acidophile Mode of adaptation
a. Membranes
- One of the adaptations that contribute to maintaining the intracellular pH of acidophiles is the impermeability of the cell membrane, which restricts the influx of protons into the cytoplasm.
- In acidophilic archaea, there is a strong association between the presence of tetraether lipids in the cell membrane and tolerance to acid pH.
- Reducing the pore size of membrane channels has been proposed as another mechanism to maintain pH homeostasis to prevent protons from entering the cells.
- Another successful modification is the incorporation of very-long-chain dicarboxylic fatty acids which make up more than 50% of the membrane fatty acid.
- These specialized mechanisms prevent the entry of protons into the cell and the acid hydrolysis of the membrane.
b. Cytoplasm
- Acidophiles maintain pH homeostasis by removing excess protons from the cytoplasm by different mechanisms.
- In the case of bacteria such as Bacillus acidocaldarius and T. acidophilum have the ability to actively pump protons out of their cytoplasm to maintain pH homeostasis, and this process is associated with the respiratory chain.
- In addition, the numerous proton-driven secondary transporters also act as an adaptation to survive at a low pH environment.
- The buffering capacity of the cytoplasm of acidophiles is also an important means of physiological adaptation of acidophiles.
- The cells contain buffer molecules that have basic amino acids such as lysine, histidine, and arginine, and these molecules help in proton removal.
c. Organic acids
- Organic acids like acetic or lactic acid function as uncouplers of the respiratory chain at low pH by diffusion of the protonated form into the cell.
- Therefore, the degradation of these organic acids might be used by heterotrophic acidophiles to prevent their harmful effect.
Acidophile Examples
- Some examples of acidophilic organisms include Lactobacillus, Thiobacillus sulfolobus, Bacillus acidocaldarius, Thermoplasma acidophilus, Picrophilus, Ferroplasma acidiphilum, Acidithiobacillus, Leptospirillum, Acidobacterium spp., Sulfobacillus, etc.
Alkaliphile Definition and Characteristics
- Alkaliphiles are a group of extremophiles that can live and thrive in environments with extremely high pH value (9-13) with the optimal pH being 10.
- Alkaliphiles are of two types; obligate alkaliphiles growing only in environments with pH higher than 9 and facultative alkaliphiles that can live both at neutral pH and alkaline conditions.
- Most of the organisms described to date as growing under very alkaline conditions are prokaryotes, comprising a heterogeneous collection of eubacteria with a few examples of archaebacteria.
- Alkaliphiles can be isolated from ‘normal’ environments such as garden soil presumably because there are transient alkaline conditions generated in such environments by biological activity.
- Alkaliphiles are believed to be the earliest life forms on Earth which originated billions of years ago in deep-ocean alkaline hydrothermal vent systems.
- Alkaliphiles thrive in many geographical locations across the planet, both in natural and manmade alkaline environments like alkaline soda lakes, soda deserts, and saline soda soils.
- Other natural habitats in which alkaliphiles flourish include the alkaline serpentine lakes, oceanic bodies, ikaite tufa columns, and alkaline hydrothermal vents.
Alkaliphile Mode of adaptation
a. pH Homeostasis
- The intracellular pH homeostasis is a vital adaptation shared by all alkaliphiles as all alkaliphiles maintain lower cytoplasmic pH than its external environment.
- Some of the most important adaptive mechanisms that play a significant role in pH homeostasis are acquiring H+ from the extracellular environment, reducing H+ leakage from the cytoplasm, production of organic acid, and deterring the diffusion of OH– from the extracellular environment.
- The monovalent cation or proton antiporters that exchange the intracellular cations such as Na+ and Li+ for the extracellular H+ are important mechanisms to increase H+ concentration in the cytoplasm.
- The ATP synthases of alkaliphiles also have a remarkable contribution to the pH homeostasis where they do not have ATPase activity, thus, preventing the release of protons out of the membrane during the transport chain.
b. Acid production
- Alkaliphiles produce metabolic acid through sugar fermentation and amino acid deaminases.
- The acid production contributes to the pH homeostasis primarily by increasing the cytoplasmic H+ concentration.
- Moreover, acid production, in addition to preventing cytoplasmic alkalinization, can increase the availability of H+ in the vicinity of the cell.
c. Cell envelope
- The cell envelope in alkaliphiles consists of secondary cell wall polymers that are rich in negatively charged residues such as aspartic acid, galacturonic acid, glutamic acid, and phosphoric acid.
- The highly negatively charged cell wall structure interacts with cations such as H+ that can delay the rapid loss of H+ from the cell surface, enhancing the bioenergetics of the alkaliphiles.
- The cell membrane of alkaliphiles consists of about 90% of branched fatty acids which help in pH homeostasis by reducing H+ leakage.
d. Damage Repair systems
- Alkaliphiles also employ different damage repair systems to maintain the necessary level of functional biomolecules, repairing the damage incurred by cytoplasmic alkalinization.
- An increase in the level of chaperone and release of protein damage repair enzymes are some of the well-studied mechanisms.
- Chaperons are known to be involved in refolding proteins that are unfolded by stresses, thus increasing the tolerance levels.
Alkaliphile Examples
- Some of the examples of alkaliphiles include Bacillus alkaliphilus, Bacillus pasteurri, Bacillus halodurans, Halobacterium, Clostridium paradoxum, Halomonas pantelleriensis, Alkaliphilus hydrothermalis, etc.
Microorganisms in extreme low humidity/water activity (Xerophiles)
Xerophile Definition and Characteristics
- Xerophiles are a group of extremophiles that are capable of surviving in environments with low availability of water or low water activity.
- Generally, xerophilic organisms are capable of growing at aw values lower than xerotolerant organisms (aw below 0.8).
- Two major types of the environment provide habitats for the most xerophilic organisms, namely foods preserved by some form of dehydration or organic solute-promoted lowering of aw and saline lakes, where low aw values are a consequence of inorganic ions.
- In environments where little water is available, organisms must take up and maintain sufficient water against extreme concentration gradients to support cellular processes.
- Xerophiles are of different types belonging to different groups of living beings. Xerophilic fungi represent a large group of xerophilic organisms.
- Eukaryotic organisms like plants capable of surviving at low water condition, called xerophytes are also xerophiles.
- Xerophiles are closely related to halophiles as halophilic environments tend to have low water activity.
- Even though water is crucial for many biomolecular processes in living beings, xerophiles have intricate means to survive in conditions with low water activity.
Xerophile Mode of adaptation
a. Dormancy
- One of the most common responses of prokaryotes to low water conditions is a reversible form of dormancy.
- These organisms under a temporary period of dormancy in the form of spores so that they reduce metabolic activity and resume normal metabolism when appropriate conditions are available.
- The formation of spores and reduction in metabolic activities provide long periods of survival for many microorganisms as well as larger eukaryotes.
b. Extracellular polysaccharides and biofilm formation
- Various xerophilic organisms form biofilms as it allows the survival of organisms in habitats with low moisture content.
- These biofilms consist of microbial aggregates and extracellular polysaccharides produced by those organisms.
- The extracellular polysaccharides in the biofilms are hydrophilic, which contributes to rapid water absorption rates and restoration of photosynthetic activity.
- Biofilm formation also reduces the need for large quantities of water as they occupy less space and have less metabolic activities.
c. Cell membrane
- The cell membrane of xerophilic organisms tends to have an increased ratio of fatty acids which creates a tighter lipid packing that preserves the membrane during desiccation.
- Increased cyclopropane fatty acid content in the membrane also reduces the membrane permeability to protons which thus, helps in balancing the intracellular pH.
- Xerophilic microorganisms adapt to low water activity by increasing the concentration of negatively charged phospholipids that facilitates the preservation of membrane bilayer structural integrity.
d. Proteins
- In the case of xerophytes, a range of proteins that counteract the effects of low water activity is produced.
- These proteins are rich in glycine and have a highly hydrophobic backbone that transitions into the ordered structure under desiccating conditions, preventing denaturation.
- Xerophilic cyanobacteria code for various shock response genes on dehydration that regulate the utilization of water in metabolic processes during desiccation.
Xerophile Examples
- Some common examples of xerophiles are Aspergillus penicillioides, Cereus jamacaru, Deinococcus radiodurans, Aphanothece halophytica, Anabaena, Bradyrhizobium japonicum, Saccharomyces bailli, etc.
Microorganisms in extreme salinity (Halophiles)
Halophile Definition and Characteristics
- Halophiles are a group of extremophiles that require high salt concentrations for their survival and growth.
- Halophiles are of two types; obligate halophiles that require NaCl concentration of 3% or more and halotolerant that survive at both average salt concentrations and higher.
- Halophilic microorganisms constitute the natural microbial communities of hypersaline ecosystems, which are widely distributed around the world.
- The general features of halophilic microorganisms are low nutritional requirements and resistance to high concentrations of salt with the capacity to balance the osmotic pressure of the environment.
- The salt requirement in halophiles is classified into three groups; low (1-3%), moderate (3-15%), and extreme (15-30%).
- Salt requirement depends on factors like temperature, pH, and growth medium.
- They are physiologically diverse; mostly aerobic and as well anaerobic, heterotrophic, phototrophic, and chemoautotrophic.
- Ecologically, the halophilic microorganisms inhabit different ecosystems characterized by a salinity higher than seawater that range from hypersaline soils, springs, salt lakes, sabkhas to marine sediments.
- These organisms are found in all three domains of life, i.e., Archaea, Bacteria, and Eukaryota.
- Halophilic bacteria are more abundant in specific phylogenetic subgroups, most of which belong to Halomonadaceae, a family of Proteobacteria.
Halophile Mode of adaptation
In order to avoid excessive water loss under high salt conditions, halophiles have employed two distinct strategies to increase the osmotic activity of their cytoplasm with the external environment, either producing compatible organic solutes or accumulating large salt concentrations in their cytoplasm to reach an equilibrium state in which the overall salt concentration within cells correlates that of the environment.
a. High salt-in strategy
- The high-salt-in strategy is another adaptation technique that protects halophiles from a saline environment in which they accumulate inorganic ions intracellularly to balance the salt concentration in their environment.
- This process involves Cl– pumps that are found only in halophiles that transport Cl– from the environment into the cytoplasm.
- Arginines and lysines are positioned at both ends of the channel to facilitate Cl– uptake, and release.
- Extreme halophiles maintain their osmotic balance by concentrating the K+ ions inside the cells.
- This is achieved by the combined action of the membrane-bound proton-pump bacteriorhodopsin, ATP synthase, and the Na+ antiporter that results in an electrical potential that drives the uptake of K+ into cells.
b. Organic salt-in strategy
- The high-salt-in strategy might be incompatible for the survival of moderate halophiles that thrive in habitats of fluctuating salinity.
- The solute-in strategy includes the evolution of inert, compatible organic solutes (osmolytes) in the halophiles.
- These osmolytes protect the microbial proteins from denaturation in the water of low salt concentrations while enhancing their tolerance to drastic fluctuations in the external saline environment.
c. Enzymes
- A high-salt environment substantially impacts protein solubility and stability and consequently, its function.
- The unfavorable interactions that disrupt internal microbial proteins caused by dehydration may be averted by modulating their net charge.
- Proteins and enzymes of halophiles have a larger proportion of glutamate and aspartate on their surfaces that result in a substantial number of protein charges and increased hydrophobicity.
- Both of these mechanisms work as a form of molecular halo adaptation of halophilic enzymes.
- Halophilic enzymes are more stable than their non-halophilic counterparts owing to their polyextremophilic characteristics.
- These enzymes remain active in high-salt environments, thermotolerant, and alkaliphilic.
Halophile Examples
Some common examples of halophilic organisms in terms of their salt requirement are:
- Slightly halophilic: Erwinia, Bacillus hunanensis, Halomonas zhaodongensis, Alkalibacterium thalassium, etc.
- Moderately halophilic: Spiribacter salinus, Halobacillus sediminis, Halobacillus salicampi, Marinobacter piscensis, Idiomarina aquatica, etc.
- Extreme halophile: Halococcus salifodinae, Halobacterium salinarum, Limimonas halophilia, Lentibacillus kimchii, Sporohalobacter salinus, etc.
Microorganisms in extreme sugar concentrations (Osmophiles)
Osmophile Definition and Characteristics
- Osmophiles are a group of organisms that are adapted to survive in environments with high osmotic pressures like high sugar concentration.
- Osmophilic organisms are similar to halophiles, and xerophiles as all of them have the capacity to survive in environments with low water activity.
- Osmophiles are mostly found in food with high sucrose content and environments with high osmolarity.
- Fungi are the most common group of organisms that survive as osmophiles. However, organisms of the group Archea and Bacteria are also important osmophiles.
- Osmophilic organisms are found in different parts of the world, especially in areas with high sugar content like food sources.
- The ability to adapt to fluctuations in external osmotic pressure and the development of specific mechanisms to achieve the adaption is fundamental to the survival of cells.
- Most cells maintain an osmotic pressure in the cytoplasm that is higher than that of the surrounding environment, resulting in an outward-directed pressure, turgor, whose maintenance is essential for cell division and growth.
- Any changes in environmental osmolarity can trigger the flux of water across the cytoplasmic membrane. Thus, osmophilic organisms develop different mechanisms to overcome the osmotic imbalance.
Osmophile Mode of adaptation
a. Osmoprotectatnts
- Osmophiles produce different osmoprotectants like alcohols and amino acids that prevent the change in osmotic pressure inside the cell.
- These solutes increase the osmotic pressure inside the cell so as to balance the turgor pressure on the cell from the outside environments.
b. Enzymes
- Proteins and enzymes in osmophiles have more protein charges and hydrophobicity that protects them against the change in the solute composition in the cytoplasm.
- The unfavorable interactions that disrupt internal microbial proteins caused by dehydration may be averted by modulating their net charge.
Osmophile Examples
- Some common examples of osmophiles include Zygosaccharomyces, Torula, Schizosaccharomyces octosprus, etc.
Microorganisms in extreme pressure (Piezophiles/ Barophiles)
Piezophile Definition and Characteristics
- Barophiles are defined as organisms that grow and thrive optimally at pressures greater than atmospheric pressure.
- The term piezophile is used as a replacement to barophile as piezo means pressure in Greek.
- Barophilic bacteria have been isolated from various deep-sea environments throughout the world and have been grown rapidly at low temperatures and high pressures.
- Bacteria living in the deep-sea display several unusual features that allow them to thrive in their extreme environment.
- Most barophilic organisms tend to be psychrophilic and thus cannot be cultured at a temperature above 20°C.
- Similarly, many barophiles tend to be obligate barophiles with few archaea acting as moderately barophilic.
- It has been seen that the pressure needed for the maximal rate of reproduction at 2°C may reflect the true habitat depth of an isolate.
- High pressure affects the survival of microorganisms, where it influences the membrane structure and functioning of the cell.
- High pressure and low temperature in deep-sea environments decrease the fluidity of lipids and even depress the functions of biological membranes.
Piezophile Mode of adaptation
a. Membrane
- High pressure might cause the formation of a gel-like membrane which then decreases nutrient uptake and processing.
- Barophiles produce increased levels of unsaturated fatty acids in the lipids and increasing the extent of fatty acid unsaturation can maintain the membrane in a functional liquid crystalline state at high pressures, low temperatures, or both.
- The reduced fluidity gives a defined structure to the membrane, which supports the normal functioning of the cell.
b. Proteins
- The high-pressure condition suitable for barophiles results in conformational changes in the proteins that inhibit their function.
- In order to prevent such changes, barophilic proteins usually have lower concentrations of proline residues and a higher concentration of glycine residues.
- The proline residues have cyclic side chains that disrupt alpha-helices, whereas the glycine residues have small side chains with high conformational flexibility.
- The increased flexibility prevents the disruption of alpha helices and protects the function of such proteins.
Piezophile Examples
- Some common examples of barophilic microorganisms are Shewanella benthica, Moritella yayanosii, Shewanella violacea, Photobacterium profundum, Moritella japonica, Sporosarcina spp, etc.
Microorganisms in rocks (Endolith/Hypolith)
Endolith Definition and Characteristics
- Endolith is an organism that survives in various inhospitable environments throughout the world, especially inside rocks, animal shells, coral reefs, and sand particles in the soil.
- Endoliths occupy habitats beneath and between porous and translucent rocks and minerals.
- Rock porosity provides interstitial spaces for microbial colonization and translucence enables photosynthesis to take place.
- Despite their limited water availability, cold temperature, strong winds, and large variations in solar radiation input, cold deserts harbor endolithic microorganisms.
- Microbial life can thrive, and endolithic microbial communities have been intensively studied in the Antarctic region, which is characterized by extreme climatic conditions, with low humidity and precipitation making it practically an inhospitable environment for living beings.
- In terrestrial systems, these microenvironments typically provide protection from intense solar radiation and desiccation, as well as sources of nutrients, moisture, and substrates derived from minerals.
- In marine systems, endolithic communities similarly exploit the rocky seafloors, but also dwell into limestone and mineralized skeleton of a broad range of marine animals.
- Besides tolerating the desiccating conditions and extreme temperatures, microorganisms inhabiting such arid conditions are subjected to osmotic stress due to the high salt concentrations.
- The water content of sandstone colonized by endolithic microorganisms is represented by 0.1–0.2% by weight as a result of little moisture penetrating into the rocks through pores.
Endolith Mode of adaptation
a. Metabolic activities
- Endoliths are capable of ‘switching’ their metabolic activities on and off in response to rapid changes in environmental conditions.
- In cold areas, the organisms are inactive in a ‘freeze-dried’ state that does not damage cellular structures.
- As the temperature increases, the small quantities of water can maintain a level of humidity that is sufficient for metabolic activity.
b. Biofilm formation
- Various xerophilic organisms form biofilms as it allows the survival of organisms in habitats with low moisture content.
- These biofilms consist of microbial aggregates and extracellular polysaccharides produced by those organisms.
- The extracellular polysaccharides in the biofilms are hydrophilic, which contributes to rapid water absorption rates and restoration of photosynthetic activity.
c. Weathering process
- Lichen and algae represent the pioneer species in such environments where the release of various enzymes results in the degradation of rock particles.
- As the weathering process continues, the organic matter of the area increases so that new groups of living microorganisms can thrive at such a climate.
Endolith Examples
- Some examples of endoliths include Leptolyngbya, Helicobacter recurvirostre, Gloeocapsa sanguine, Acaryochloris, Chroococcidiopsis, Anabaena, Spirirestis rafaelensis, etc.
Hypolith Definition and Characteristics
- Hypoliths are organisms or communities of organisms that live on the underside of rocks or at the rock–soil interface.
- Hypoliths are photosynthetic microorganisms that exist in hot and arid climates, usually at the interface between the rocks and the soil.
- The community of microorganisms present in such an area is termed as hypolithon.
- Microorganisms that are present underneath the rocks are protected from the harsh radiations of the sun and the wind.
- The rocks might even trap moisture which can then be used by these microorganisms.
- Different forms of minerals like quartz are found in soil and rocks that also supports different forms of life.
- However, there are different stresses, including low water activity and drastic changes in temperature, which limits the biodiversity of such habitats.
- The most common habitats for hypoliths include the desert lands and polar regions where the climate change is quite drastic with rapid desiccation and rehydration.
Hypolith Mode of adaptation
a. Biofilm
- One of the most successful means of survival of microorganisms in rock surfaces is by the production of exopolysaccharides that together microbial aggregates form biofilms.
- Biofilms help to retain moisture and also reduce the metabolic activities of these organisms, which help to save energy and nutrients.
- Biofilms also protect hypoliths against the harmful radiation and extreme heat conditions of such habitats.
b. Dormant stages
- In the case of low water activity, some of the hypoliths modify into dormant stages in the form of spores that help to preserve nutrients and moisture of the surface.
- The dormant stages then convert into the active forms as the climate and nutrient content of the environment becomes sufficient.
- The formation of spores and reduction in metabolic activities provide long periods of survival for many microorganisms as well as larger eukaryotes.
Hypolith Examples
- Some of the common examples of hypoliths include Nostoc, Bryum, Hennediella, Stichococcus mirabilis, Ichthyosporea, etc.
Microorganisms in heavy metals (Metallotolerant)
Metallotolerant Definition and Characteristics
- Metallotolearnt microorganisms are the microorganisms that are capable of tolerating and detoxifying high levels of dissolved heavy metals.
- Microorganisms utilize metals as structural components of biomolecules, as cofactors in reversible oxidation/reduction reactions, and in electron transfer chains during energy conservation.
- However, metals can become toxic if their intracellular concentrations are too high.
- Most metallotolerant microorganisms tend to be acidophilic as the physiological activities of such microorganisms enable tolerance against high metal concentrations.
- As many metals are more soluble at acidic pH, acidophiles are typically exposed to high metal concentrations and can survive in 1000-fold higher amounts than neutrophilic microorganisms.
- Metallotolerant microbes belong to all bacterial groups studied, mostly among aerobic and facultative aerobic chemo-heterotrophic microorganisms.
- Polluted soils and waters with untreated industrial and urban wastes and samples of the natural environment with a high concentration of metals are important habitats of metallotolerant microorganisms.
- These organisms have different mechanisms that support their survival in very high metal concentrations.
Metallotolerant Mode of adaptation
a. Membranes
- The membranes of metallotolerant microorganisms are equipped with various channels that prevent the entry of metals ions into the cytoplasm.
- A process termed metal sorption is common in metallotolerant microorganisms where the metal ions are bound to the membrane but cannot enter the cell.
- Besides, different transport channels have regulators lie the ferric uptake regulator that regulates the entry of a large number of iron ions into the cell.
b. Mineralization
- The process of conversion of organic compounds into inorganic forms so that they can be excreted out of the cell is called mineralization.
- The process is common in many metallotolerant, where they have different enzymes that catalyze the mineralization process.
- Other metabolic processes that convert toxic forms of metals into less toxic forms via enzymatic oxidation and reduction are also studied in these organisms.
Metallotolerant Examples
- Some of the common examples of metallotolerant species include Bacillus subtilis, Bacillus megaterium, Acidithiobacillus ferrooxidans, Acidithiobacillus caldus, Corynebacterium diptheriae, Acidiphilium rubrum, Acidiphilium multivorum, etc.
Microorganisms in extreme Radiation (Radiophiles)
Radiophile Definition and Characteristics
- Radiophiles are a group of extremophiles that are capable of surviving extreme forms of radiations like ionizing radiant (gamma rays) and UV radiation.
- Studies on radiophiles are quite limited as they are to be isolated from extreme environments like outer space of other planets.
- These organisms have low diversity with all organisms belonging to the archaea and bacteria families.
- Radiophiles can either be radiation tolerant or radiation-resistant. Radiation tolerant microorganisms can endure harmful radiation for a period of time, whereas radiation-resistant organisms can withstand a longer period of time.
- Radiations are harmful to neutrophils as they destroy various important biomolecules like DNA, proteins, and enzymes as a result of ionization.
- Non-ionizing radiation, in turn, results in the formation of reactive oxygen species like superoxides which then affects the metabolism of those cells.
- The adaptive mechanism utilized by radiophiles might be different for ionizing and non-ionizing radiation.
Radiophile Mode of adaptation
a. Ionizing radiation
- Ionizing radiation is responsible mainly for double-stranded breaks in the genome of organisms.
- However, it has also been shown to damage both proteins and lipids and induce persistent oxidative stress.
- Therefore, ionizing radioresistant organisms have developed all, or a combination of different strategies like the novel and adaptive DNA repair mechanisms, antioxidant and enzymatic defense systems, and a condensed nucleoid.
- Fast and accurate repair of genomes is essential in surviving doses of ionizing radiation which is accomplished in radiophiles through the use of the nucleotide excision repair pathway.
- Other forms of oxidative stress prevention and tolerance mechanisms include cell cleaning through the elimination of oxidized macromolecules, selective protection of proteins against oxidative damage, and suppression of reactive oxygen species production.
- A condensed nucleoid has also been shown to promote the efficiency and accuracy of DNA repair and to limit the diffusion of radiation-generated DNA fragments.
b. Non-ionizing radiation
- UV radiation, unlike gamma radiation, damages DNA in more subtle ways through the formation of cyclobutene pyrimidine dimmers.
- To repair these DNA lesions, organisms use a combination of photoreactivation genes, nucleotide excision repair, base excision repair, and homologous recombination.
- These organisms have also developed a suite of photoprotection devices to protect themselves from continual exposure to UV radiation.
- Products like carotenoids, superoxide dismutases, and hydroperoxides and the gene duplication process via polyploidy work as the photoprotection devices.
- The genome composition of the organisms with a reduction in the number of bipyrimidine sequences also provides protection against exposure.
Radiophile Examples
- Some common examples of radiophiles are Deinococcus radiodurans, Brevundimonas, Rhodococcus, Halomonas, Herbaspirillum, Hymenobacter, Rhodobacter, etc.
Applications of Extremophiles
- Exremophilic enzymes have been model systems to study enzyme evolution, enzyme stability, activity, mechanism, protein structure, function, and biocatalyst under extreme conditions.
- Thermophiles have yielded stable α-amylase for starch hydrolysis, oxylonases for paper bleaching, and proteases for brewing and for detergent purposes.
- Alkaline active proteases, amylases, cellulases, mannanases, lipases, etc. are used in the formulation of heavy-duty laundry and dishwashing detergents as they are efficient in removing stains and allow effective low-temperature (30–40°C) washing.
- Some species of acidophilic microorganisms can be used not only to reduce mine water pollution but also to recover metals from acidic wastewater via selective biomineralization.
- Extroenzymes like Taq polymerase from Thermus aquaticus is an ideal for use in a polymerase chain reaction as it reduces the need for adding extra polymerase during the reaction.
- Cellulose for various extremophilic organisms has been used for the treatment of juices, color brightening in detergents, and treating cellulose-containing biomass and crops to improve their digestibility and nutritional quality.
- Similarly, halophiles are being exploited as a potential source of carotene, compatible solutes, glycerols, and surfactants for pharmaceutical use.
- Some extremophilic microorganisms may also comprise a large reservoir of novel therapeutic agents—for example, iron-binding antifungal compound, pyochelin isolated from halophilic species of Pseudomonas.
- A thermostable glucokinase from the thermophilic species, Bacillus stereothermphiolus, can be used as a glucose sensor for quick glucose assay.
- Alkaline active enzymes have got several notable applications in textile and fiber processing in processes like cotton scoring and blast fiber degumming.
- Alkaliphiles and their enzymes have been tried in various synthesis reactions with peptide synthesis being the most important one.
- Information on the microbial composition and biogeochemical cycling of extreme ecosystems also helps in understanding the global change, threats, and opportunities for living beings.
- Enzymes from extremophiles can also be used in bioremediation processes like toxifying wastewater and air and removing metallic waste from sewages and industries.
- Different barophilic enzymes are used for the production and sterilization of items at varied pressure conditions.
0 Comments